Microscale techniques
In order to understand, and alter, the behaviour of a material in a fire, it is necessary to know as much as possible about the processes of decomposition.
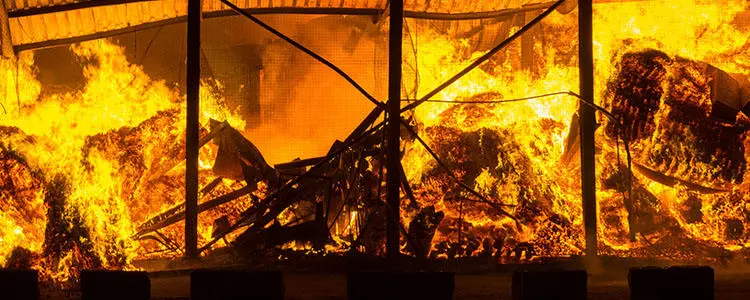
These processes are often highly dependent on the conditions, particularly the atmosphere. The sensitivity of changes to say air, nitrogen, air/nitrogen, and even oxygen, or to heating rate or sample shape and size, can help to identify the processes occurring. Moreover, sensitivity to these parameters gives away vital clues needed to interpret the fire behaviour.
Our equipment
Microscale Combustion Calorimetry (MCC) (ASTM D7309)
This technique was developed at the fire laboratories of the Federal Aviation Administration in the U.S. and measures the heat of combustion of products evolved during programmed heating. This directly links microscale thermal analysis and flammability properties. MCC evaluates the combustibility of milligram-scale (generally 1 – 3 mg) samples. The components of the apparatus are shown schematically below.
The sample is placed in an inert, heat-resistant crucible (often ceramic) and transported to the specimen chamber. Here, it is heated under a controlled temperature programme (1 K/s) in an inert atmosphere. As the sample is pyrolysed, volatile products are released, which rise into the combustion mixing section - a chamber where volatiles are rapidly mixed with oxygen at 900 °C. Oxygen combustion calorimetry is used to calculate the heat of combustion of the pyrolysis products, and a computer algorithm converts the oxygen depletion and mass flow rate data into specific heat release (W/g) as a function of temperature. Curve fitting software can be applied to obtain values for heat release capacity and total heat release. Information on the peak of specific heat release rate and temperature of the peak of specific heat release rate is also useful for evaluating the fire performance of materials. The MCC apparatus has gained popularity thanks to its effective design, relatively low cost and ease of use. It is particularly popular with the aircraft manufacturer, Boeing, who uses it for material screening and quality control.
Thermogravimetric Analysis (TGA) (ASTM E1131, BS EN ISO 11358-1)
Thermogravimetric Analysis provides the crucial material property data with which to interpret the complexities of burning behaviour. For example, ignition can only occur when there is sufficient fuel present in the gas phase. The temperature at which the first sharp mass loss occurs normally corresponds to the critical surface temperature for ignition, and if the addition of fire retardants delays this onset, they may also delay ignition.
A sample is suspended on a highly sensitive balance over a precisely controlled furnace. Usually heating rates of 10 – 20°C/min are used to look for broad decomposition stages, while slower heating rates around 1°C/min are better for isolating individual events. Decomposition in air indicates the processes which may occur before ignition, while their absence or delay under nitrogen is indicative of a condensed phase decomposition mechanism. Sample sizes are usually kept as small as possible, within the limits of the apparatus this is usually around 5 mg per run. This reduces bulk effects and at higher heating rates avoids thermal gradients being set up within the sample. Our instruments allow for heating rates of up to 100°C/min and temperatures up to 1000°C. In addition, the design allows for purging of corrosive gases and for rapid gas changes. For example, the carbon content of a residue may be determined by changing the atmosphere to oxygen at 800°C.
Differential Thermal Analysis (DTA) and Differential Scanning Calorimetry (DSC) (ISO 11357)
Differential Thermal Analysis (DTA) and Differential Scanning Calorimetry (DSC) (ISO 11357) allow quantification of the energy required, or released, during temperature-programmed heating. Differential Thermal Analysis (DTA) and Differential Scanning Calorimetry (DSC) (ISO 11357)In differential thermal analysis (DTA), the temperature of the sample is compared to the temperature of a reference (usually a matching empty crucible) during programmed heating (or cooling). Raw DTA data may be converted into energy units, to make the results comparable to conventional differential scanning calorimetry (DSC). It is recognized that both techniques provide the same information and are both classed as DSC. Data is reported as a differential, showing endothermic (heat to sample) or exothermic (heat from the sample) processes. As the most commonly used thermal analysis technique, DSC also provides the following quantitative measurements of physical processes and characteristics of polymers.
Thermophysical Properties:
Specific heat capacity
Product Identification and Characterization:
Melting temperatures
Transition enthalpies
Phase transformations, phase diagrams
Crystallization temperatures
Degree of crystallinity
Glass transition temperatures
Advanced Material Analysis:
Decomposition and oxidative susceptibility
Reaction kinetics
Purity determinations
Simultaneous Thermal Analysis-Fourier Transform Infrared (STA-FTIR)
This technique combines the benefits of thermogravimetric analysis (TGA) and differential scanning calorimetry (DSC) (measurement of the energy flow to or from the sample, quantifying the changes as exothermic or endothermic) into a single experiment.
The instrument also has a maximum temperature range extending to 1500°C. Although the enthalpy changes are only semi-quantitative, it has the great advantage of allowing precise correlation of events resulting from mass loss with enthalpy change. Thus if an exothermic process just precedes material loss, the sequence of events can be clearly known.
Gas phase Fourier Transform Infrared Spectroscopy (FTIR) (ISO 19702)
Gas-phase Fourier Transform Infrared Spectroscopy (FTIR) (ISO 19702) has revolutionised infrared analysis, producing spectra of higher resolution in a much shorter time. For polymer decomposition studies this allows the gas-phase products to be collected continuously as a function of temperature. Gas-phase infrared spectra are much sharper than condensed phase spectra, and generally recognition of the individual molecules is possible in the gas phase, whereas only functional group identification is normally obtained from condensed phase spectra.
STA coupled together with the gas phase FTIR provides a very rich profile of the spectra of all the evolved fire effluents and can be then deconvoluted to provide profiles with respect to temperature of the evolution of individual species. This gives a complete profile of the processes occurring during decomposition.
Hyphenated Thermogravimetric Analysis - Fourier Transform Infrared - Gas Chromatography (TGA-FTIR-SRA-GCMS)
Hyphenated Thermogravimetric Analysis - Fourier Transform Infrared -Gas Chromatography (TGA-FTIR-SRA-GCMS) analysis is the most powerful and complete approach to investigate material decomposition. This combination will not only lead to characterise primary components of the tested product but also allow to identify additives or contaminants. It can also provide the qualitative and quantitative analysis of evolved gases.
Produced volatiles in the TGA furnace are transferred to the FTIR gas cell where spectra are produced. Then hot gases will be further flowed to the SRA storage interface which allows to store up to 16 loops of the gaseous effluents from FTIR and to automatically inject them into the GCMS.
Pyrolysis-Gas Chromatography-Mass Spectrometry (py-GCMS)
Pyrolysis-Gas Chromatography-Mass Spectrometry (py-GCMS). In order to understand the decomposition of a polymer or the mode of action of a fire retardant, it is normally necessary to understand the chemistry behind the real-scale observations. This is a simple controlled temperature pyrolysis experiment, which allows volatile pyrolysis products to be analysed by Gas Chromatography (GC), GC-MS.
Pyrolysis-Gas Chromatography-Mass Spectrometry (py-GCMS). Pyrolysis GC-MS complements TGA-FTIR as it provides a snapshot identifying all evolved species over a particular temperature range. Samples can be decomposed in the air or inert gas, at heating rates from 1°Cmin-1 to 1000°C min-1, either replicating the decomposition conditions in a thermogravimetric analyser, or the more rapid heating rates of a real fire. This provides a snapshot of the species present at a particular stage in the decomposition.
In the example below, a copolymer of soya oil, styrene and divinylbenzene (SSD) was decomposed in nitrogen at 10°C min-1 and the volatiles was collected between 50-500°C.
Rheology (BS EN ISO 3219)
Rheology (BS EN ISO 3219) is designed to quantify the gel-like or viscous behaviour of a polymer in the liquid state. The term rheology (from rheo meaning “to flow”) refers to both physical deformation and flow of material under an applied force. Thus, the rheological behaviour of polymers encompasses a wide range of macroscopic phenomena including the flow of viscous liquids, the mechanical properties of elastic solids, and viscoelasticity (the time-dependent mechanical properties of a polymer).
The polymer sample is mounted between two parallel circular metal plates. One plate is driven mechanically with an oscillating motion of fixed angle or torque, and the resistance to the motion is recorded. A decomposing thermoplastic changes from a fluid-like or gel-like solid, to a gel-like or viscous liquid often with no distinct transition from one phase to the other. Various parameters including the elastic and relaxation modulus and the viscosity can be determined. The complex dynamic modulus G* can be separated into two components, the storage modulus G’ (representing the solid or gel-like behaviour) and the loss modulus G’’ (representing the viscous or liquid-like behaviour).